Internet of Things (IoT) is today’s megatrend in wireless communication industry. Machine to machine wireless connectivity is being adopted in various verticals at increasing pace. Number of IoT devices deployed by 2020 is expected to increase to ~20 Billion.
Cellular IoT (CIoT) is defined as set of technologies under the 3GPP umbrella to enable IoT connectivity using the licensed frequencies, co-existing with the legacy cellular broadband technologies such as LTE, UMTS and GSM. The main CIoT technologies being rolled out globally are NB-IoT, and LTE Cat-M1. The term CIoT is used to make the distinction to non-3GPP IoT technologies such as LoRa and SigFox, which are deployed in unlicensed bands.
IoT applications can be categorized to massive IoT and critical IoT. Massive IoT includes smart metering, home security, etc. The requirements for massive IoT include years of battery life, scalability to very large number of devices, robust coverage and deep indoor facilities. NB-IoT technology is optimized for this use case. Critical IoT includes applications such as health care and connected car, where very low latency levels on ultra-reliable networks, often combined with very high throughput is required. LTE Cat-M1 is optimized to meet these requirements.
LTE Cat-M1 | NB-IoT | |
Heritage | LTE | Clean-slate |
Bandwidth (downlink) | 1.4 MHz | 180kHz (12 by 15kHz) |
Bandwidth (uplink) | 1.4 MHz | Single-tone (180kHz by 3.75kHz or 15kHz) or multi-tone (180kHz by 15kHz) |
Multiple access (downlink) | OFDMA | OFDMA |
Multiple access (uplink) | SC-FDMA | Single-tone FDMA or multi-tone SC-FDMA |
Downlink peak bitrate | 1Mbps | 250kbps |
Uplink peak bitrate | 1Mbps | 250kbps(Multi-tone)
20kbps (single-tone) |
Latency | 10ms-15ms | 100ms ~8s |
Coverage (link budget) | ~156dB | ~164dB |
Mobility | Full | Nomadic |
Applications | Critical IoT: health care, home care, traffic SoC, connected car, etc. | Massive IoT: smart metering, home security, etc. |
Cellular IoT Field Testing – Verifying the Real Performance
The emerge of IoT poses various challenges for the network operators, as well as to industrial customer verticals and M2M connectivity providers. What level of QoS and SLAs can be achieved and committed? Are there any differences in performance between network equipment manufacturers, IoT device manufacturers, and different operators? How should the network be configured and planned to achieve optimal performance? Is the service coverage network wide, including deep inbuilding? How long is the battery life of my IoT device? Answers to these questions are dependent on the field performance of the commercial network equipment and IoT devices, interoperability between the equipment, and the network design and configuration. The 3GPP specification provides boundaries for the performance and operations of IoT, but the equipment implementations and the network design play a major role on the real field performance. Hence, field measurements are needed to obtain definite answers.
Specifications vs. Commercial Implementations
The 3GPP standard defines the technologies to deliver the key features of IoT, but many of the details on how these technologies are used and implemented are proprietary and vendor-specific. Further the device antennas and housing may have significant impact in the practical performance achieved. Hence, testing and benchmarking of network equipment manufacturers as well as the devices available in the market is crucial to verify the interoperability and performance of the equipment to be deployed. In this initial field/lab testing, tools are needed that will provide a holistic view from application level down to RF and control of plane signaling.
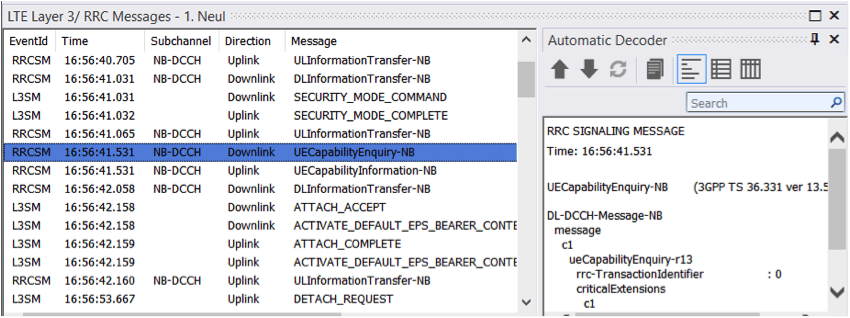
Signal coverage
IoT is designed to offer better coverage compared to existing mobile broadband technologies. Maximum coverage gain over regular LTE is expected to be 23dB for NB-IoT and 15dB for LTE Cat-M1. The real gain may be less, depending on deployment method and configuration. Increased coverage enables operators to deploy cellular IoT technologies in the existing LTE base station grid, achieving a “deep inbuilding” coverage without, or with reduced additional indoor cell and DAS deployments. Target is to provide sufficient coverage for smart meters and other IoT appliances that are typically located in basements and similar deep inbuilding locations.
Since IoT coverage is better than with any of the existing cellular technologies, and the existing base station grid is designed to provide sufficient coverage for established cellular broadband technologies such as LTE, no major coverage issues are expected in outdoor environments when IoT is deployed in the existing base stations. When the IoT coverage is verified via measurements, focus must be in deep-inbuilding to stress the system to the designed performance limits, and to gather an understanding on how “deep” the indoor coverage really is.
Service coverage
Another important point to consider when testing NB-IoT is service coverage. Determining the IoT coverage is not simply a matter of signal strength and block/bit error rate. Coverage in both NB-Iot and LTE Cat-M1 is improved mostly with the expense of latency, bandwidth, and spectral efficiency. One of the key techniques used is repetitions, that is, redundant transmissions of the same frame multiple times, and combining the repeated transfers in the receiving end. Every repetition increases the coverage by 3dB assuming ideal channel estimation and TX/RX chain. However, latency experienced by the application is doubled, throughput and spectral efficiency are sliced to half. As a result, round trip may be increased up to ~10s in extreme low coverage. Similarly, throughput decreases down to range of 100bps in extreme low coverage.
As discussed, spectral efficiency is also decreased drastically in extreme low coverage. This easily goes unnoticed at the early stages when IoT networks are not yet capacity limited, but will have a big impact down the road when the IoT technologies are widely adopted and the number of users in the cell increase to thousands. Seemingly small difference in the number of repetitions needed has huge impact in the number of IoT users that can be accommodated in a cell.
It is important to first establish minimum accepted QoS in terms of latency and bitrate, and then verify the coverage against that boundary. This must be kept in mind also when benchmarking performance of network equipment and/or IoT devices. All equipment may reach the extreme coverage, but with very different QoS.
Figure 2.Cat-M1 service coverage (Round Trip Time) verification results from live network active tests using Keysight’s Nemo Analyze measurement post-processing toolThe only way to verify the service coverage accurately is with active tests with an IoT device connected to measurement tool SW where a test protocol such as Ping is used to generate load over the connection between the device and the network. The measurement application collects the application performance readings, as well as the radio metrics by tapping into the diagnostics interface of the IoT modem/chip of the device.
Outcome of the service coverage test is minimum signal coverage and quality threshold for achieving acceptable QoS. Once the thresholds are known, network scanners can be used to measure the signal coverage and quality on wider area more efficiently. Scanner can simultaneously measure the signal strength of all cells, from all operators, making it possible to assess the coverage accurately enough for optimization, troubleshooting, and benchmarking purposes. The scanner results can be then mapped to service coverage results.
IoT devices are typically measuring only serving cell quality and coverage during active connection, not neighbor cells. In NB-IoT, this is because the standard does not support seamless mobility and handover. In LTE Cat-M1 handover is supported by the 3GPP specification, but not configured in all live networks due to power saving reasons. Hence IoT devices cannot be used to collect signal coverage and quality readings comprehensively in drive testing and scanners will be needed for the purpose. On the other hand, most important to start with is to perform active tests using IoT device to verify the service coverage. Also, IoT testing is expected to focus on inbuilding, where stationary and low mobility testing is performed, and scanner measurements are less important.
Battery life and power consumption
Power consumption of IoT devices is another important factor, directly linked to the coverage as well as to network design and configuration. Both LTE Cat-M1 and NB-IoT are designed to significantly decrease power consumption over broadband cellular technologies. The key technique of power saving is DRX in (Discontinuous reception) in a few different flavors. The principle in these techniques is that the modem is effectively switched of during the idle periods. In NB-IoT, the maximum battery life expected is in the range of 10 years in applications where small data packets are send sporadically. The battery life is important for many low cost, unmanned sensor applications of IoT. Ideally the battery should last for the whole life cycle of the device to avoid costly maintenance. Coverage has a big impact on the battery life. In low coverage, more repetitions are needed to successfully transfer the data. The more repetitions, the longer the duty cycles of the IoT modems, and higher the power consumption respectively. Excess repetitions due to network misconfiguration or network implementation also have a similar impact. In a given deep inbuilding location, different operators may have difference of tens of dBs in the coverage. This can result to years of difference in the maximum battery life of IoT device deployed in the location depending on operator. Hence coverage remains to be an important benchmark KPI between operators.
Summary
Cellular IoT performance is heavily dependent on IoT devices, network equipment implementation, interoperability between the equipment, and network design. Field measurements are important for operators and industrial IoT customers to verify and benchmark the performance of IoT solutions.
Enhanced coverage is one of key features of Cellular IoT. The coverage boost is achieved with the expense of quality of service (QoS). Hence, active testing and verification of both QoS and signal coverage are needed to understand and benchmark the true service coverage. Coverage has also direct impact to the network capacity and device power consumption.
Keysight Network Testing develops and sells RF measurement and analysis equipment under the Nemo brand. Nemo Outdoor drive test solution has been used by operators in multiple pilots and coverage measurements in IoT networks.
Register for the upcoming Keysight Webinar “NB-IoT and LTE CAT-M1 – RF measurements for CIoT (Cellular IoT)“
About the author:
Matti Passoja, Director, Post-Processing Products at Keysight Network Testing. Matti is responsible for solution marketing at Keysight Network Testing (NT). This includes sales/channel enablement, outbound marketing, Go To Market (GTM) plan: Further Matti has 10+ year experience in R&D and product management of data collection and analytics