
Only exclusive licensing of additional mid-band spectrum for full-power use can provide sufficient radio propagation and received-signal strength to deliver the increased cellular data capacity required across entire national networks. Alternatives, including low-power unlicensed, medium-power shared and millimeter wave spectrum that require extensive network densification with small cells are costly and uneconomic beyond usage hotpots and limited areas indoors where such implementations are well suited.
Cellular network data traffic has on-average doubled every couple of years since mobile broadband took off in the late 2000s with introduction of HSDPA, then LTE — driven by mass-market adoption of PC dongles and then smartphones. That growth rate has even still occurred over recent years in the US, despite already relatively high usage levels with abundant 5G availability. Exponential rate traffic growth will continue for many years.
2024 hindsight
A vision for 1,000x data traffic growth was inspired by demand for mobile broadband and strong data traffic growth over the first couple of years following commercial LTE service launches from yearend 2009. It was rightly presumed that demand for mobile data would escalate exponentially so long as network capacity was provided cheaply enough with supply growth fueled by a combination in the triumvirate of three factors:
- Additional spectrum
- Improved technologies
- Network densification
An article entitled “2020 vision for LTE” that 3GPP commissioned me to publish on its web site in 2012 reflected discussions at a 3GPP Radio Access Network technologies Technical Specification Group workshop session that year. This was several years before there was anything like a commitment to or naming of 5G New Radio. That term was not officially introduced until 2016.
A contribution to the workshop by SK Telecom graphically and quantitatively depicted how network performance could be improved by the three factors above to massively increase data throughput capacity.
A 2012 view of how to enable 1000x cellular data growth
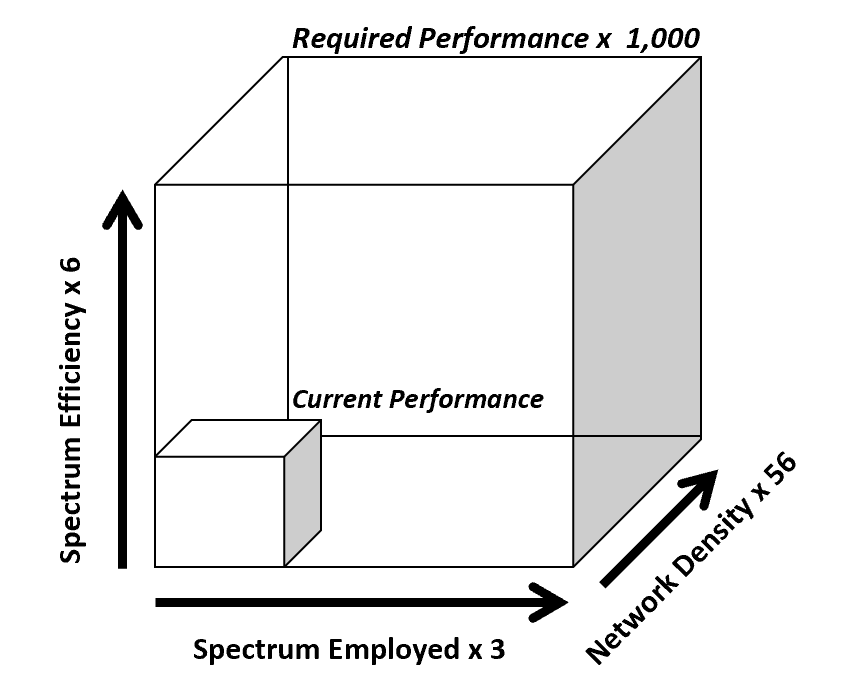
It was envisioned in the above that the increase in data capacity performance could be achieved by the multiplicative product of these three compounding improvements: 3 x 6 x 56 = 1,008.
SK Telecom’s overall prediction that 1,000x could be achieved was correct. I also thought that much growth, versus 2010 figures, was feasible and likely eventually, but I had already concluded it was not going to be achieved by 2020. Instead, in a forecast I published in 2011, I predicted that multiplier would be achieved over the 15 years from 2010 to 2025. The following graph uses a logarithmic scale to compare forecasted versus actual data traffic over three orders of magnitude of growth. It shows that my exponential forecast, made in 2011, has remained in remarkably good agreement with the huge actual mobile data traffic growth, as measured in Ericsson’s Mobility Reports, over that long time span.
Global cellular network data traffic — forecast to 2025, actual figures to 2024
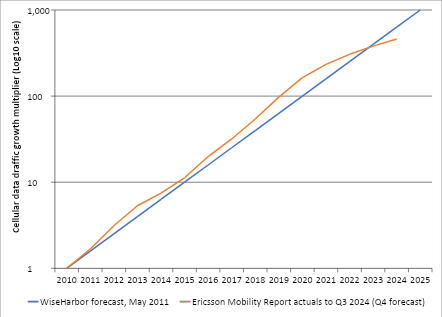
Global cellular data traffic growth rates exceeding 50% annually over many years have faltered somewhat over the last year or two. Nevertheless, the 1,000x goal will likely be reached within a few years, and then soon significantly exceeded with continuing exponential growth in demand for mobile broadband.
The spectacular capacity growth satisfying that demand is akin to what has also been achieved over decades in silicon chips with performance doubling every couple of years, as predicted by Moore’s Law.
Same ingredients, various definitions and proportions provide similar overall result
Capacity growth is arguably being achieved with somewhat different proportions among the three different types of improvements envisioned and predictively quantified by SK Telecom in 2012. Whether it got its predictions right for each of the three factors depends on definitions. In my article for 3GPP back then, I also anticipated “The addition of many small cells in HetNet configurations including macro, micro, pico, femto, relay stations and even clouds of antennae will provide the biggest boost to capacity through extreme frequency reuse.” However, while the addition of small cells has been a boon in the relatively small areas where people gather en mass—such as in sports stadiums, music arenas, transport hubs and visitor hotspots—versus a nation’s total landmass, these additions have had only modest uplift on the ability of networks to accommodate total data traffic growth demanded network-wide. Few people spend more than a very small proportion of their time or use more than a small proportion of their data in such places. Densification with additional macro cell sites or small cells across entire national networks is very limited. On the other hand, increased spectrum reuse—the defining characteristic of any cellular network—is now being achieved virtually via beamforming with massive MIMO.
The following descriptions include what is possible and what has actually been deployed. For example, while there is some 5G deployed in many nations, it is typically not yet available network-wide or accessible to all users, many of whom do not yet have 5G devices. Capacity increases so far are a blended average, for example, including 4G and 5G, with and without massive MIMO. There’s still some way to go yet before deployed capacity has been increased one thousand-fold, as indicated graphically above.
More cells
Even with the addition of small cells, physical network densification with additional end points has increased much less than that predicted in SK Telecom’s 2012 workshop contribution. Most cell sites had already been sectorized by then. Estimated numbers of small cells vary significantly, depending on classification. However, according to the Wireless Infrastructure Association, in the US at the end of 2022:
- 142,100 cellular towers were in operation;
- 209,500 macrocell sites, not including small cells, were deployed;
- 678,700 macrocell sectors, not including small cells, were in operation;
- 452,200 outdoor small cell nodes were in operation; and
- 747,400 indoor small cell nodes were in use, including private CBRS networks, DAS, small cells and mmWave and other licensed frequency bands
Even assuming few small cells at the outset around 2012, given that there has been no great proportionate change in the number of macrocell sites over the years (e.g. fewer than 25% more in the US by any reckoning) the overall densification factor is still no more than 6 rather than 56. Other estimated cell counts are appropriately even lower, probably because, for example, they exclude indoor private networks. Verizon has reportedly approximately 67,000 macrocells and only around 30,000 small cells. At yearend 2022, T-Mobile USA reportedly had 79,000 macro cell sites and 41,000 small cell and distributed antenna system sites.
Consolidation among mobile network operators significantly reduces the total number of cell sites. For example, within around two years of T-Mobile’s acquisition of Sprint closing in 2020, T-Mobile had decommissioned 30,000 redundant macro cells. Combining or deploying additional spectrum resources on fewer sites reduces densification.
Enterprise network private small cells account for the higher figures above, but these by definition are not adding to public network capacity. Home femtocells don’t really densify because these are deployed to extend coverage rather than to increase capacity with frequency reuse. Moreover, they have failed to obtain much market acceptance. Some nations might have more densification than the US, but it seems very unlikely that the average level of densification worldwide including in developing nations such as populous India, Brazil and Indonesia would exceed that of the US.
The private network case study presented online at RCR Wireless’ Industrial 5G Forum recently by Robert Greiner, Global Manufacturing Network Manager at food manufacturer Cargill, revealed how the fewer “antennas” required in cellular can be more cost effective than the much denser deployments required using WiFi access points. He said that despite 5G equipment being more costly per antenna, total equipment costs are similar because WiFi requires three to four times as many access points indoors. However, 5G is much cheaper to install—initially, and in 4-5 years when it will likely be time for lifecycle upgrades. This is because installation costs per end point are similar for 5G and WiFi, but with only one third as many needed for 5G. Outdoors, “5G shines” with the possibility of “lighting up” an entire facility with one or two antennas, he said. Furthermore, 5G equipment costs are decreasing.
While the above case study is a private network, it also suggests why low-power implementations, with WiFi or LTE-LAA in unlicensed spectrum, also provide less economic public network coverage than full-power cellular beyond traffic hotspots.
More bands
According to the FCC’s CMRS report, paragraph 276, published in June 2011, cellular spectrum potentially usable for mobile wireless services was primarily low-band (below 1 GHz) and mid-band (up to 6 GHz), with a total of 691.5 MHz of licensed bandwidth.
FCC Chairman Genachowski, back then, recognized a “spectrum crunch.” He and his successors have worked to successfully free up more spectrum for mobile broadband including its reallocation from other uses, such as cable TV distribution via satellite, despite vigorous lobbying against that by broadcasters.
According to the FCC’s December 2022 Communications Marketplace Report, paragraph 83, there was 204 MHz of low-band bandwidth and a total of 1,123 MHz of bandwidth exclusively licensed for cellular use in the US, including mid-band with 280 MHz of that in the C-band (around 3.7 GHz). According to Analysys Mason in October 2022, the average amount of mid-band spectrum among leading nations making that bandwidth available including Canada, China, Japan, South Korea and the UK was set to increase to 660 MHz by the end of that year.
The ITU’s WRC-23 meeting in late 2023 identified additional mid-band spectrum in the upper 6 GHz band (6.425-7.125 GHz) for mobile use by countries in every ITU Region worldwide. According to the GSMA, this band is now harmonised for expanding mobile capacity for 5G-Advanced and subsequent technologies with support from countries representing over 60% of the world’s population. Also according to the GSMA, this will provide 700 MHz of bandwidth.
According to 5G Americas, a “new spectrum block at 7.125 – 8.400 GHz is important for 6G radio deployments for providing 10-20 times more capacity and higher data rates while reusing existing base station sites.”
Based on these increases and their timings, SK Telecom’s projected tripling of spectrum bandwidth employed when 1,000x is achieved was not a bad estimate, but has not yet been reached. Based on Ericsson Mobility Repot figures, as analyzed in the tracking of those against my forecast, the global data growth multiplier from a 2010 base year to 2024 (extrapolating most recent growth to yearend) is 460x.
However, if millimeter wave is also included, the spectrum bandwidth multiplier contributing to the above would seemingly be many times higher in those nations that have licensed those higher-frequency bands. In the US, a total of 5 GHz of bandwidth has been licensed for cellular across the 24GHz, 28GHz, 37GHz, 39GHz and 47 GHz bands. While that corresponds to more than seven times more total bandwidth being available than in 2011, it would be incorrect to infer that all this is being or could ever be economically “employed” broadly, on a border-to-border or coast-to-coast national basis as is low-band and increasingly mid-band spectrum.
The major limitation with millimeter wave is that it is only cost effective to deploy it on a focused geographic basis. It’s great for sports stadiums, music arenas, transport hubs and in busy places like shopping malls and Times Square in New York City, but it is not viable for increasing mobile network capacity on even a moderately broader basis due to its limited signal propagation characteristics. Signals attenuate much more than lower frequencies over distance and through obstructions including concrete walls, energy-efficient windows and foliage. Consequently, a higher bandwidth increase multiplier is only applicable in a very small proportion of the US landmass, and so should be significantly discounted when estimating overall practical potential nationwide and globally.
Getting more out of each and every Hz
New and improved technologies have also been most significant in achieving the increased capacity required to accommodate data traffic growth. Enhanced technologies, for example, in modulation, have increased spectral efficiency (i.e. number of bits per Hz) within radio channels. Channel coding with improved error detection and correction also increases data throughput. The addition of carrier aggregation improves trunking efficiency.
Technological improvements and updated standards have also enabled much more spectrum to be accessed by cellular. Spectrum bands that were never conceived to be viable for 3G and 4G are being used by 5G. For example, 5G is the only cellular standard that can access mid-band frequencies approaching 4 GHz and higher. Some of these bands, including C-Band (3.7 GHz), have become mainstays for 5G. While Sprint held at least 120 MHz bandwidth of 2.5 GHz spectrum nationwide in which 4G technologies (first WiMAX and then LTE) were deployed for many years, it has only been in the last five years that this spectrum has become regarded as prime “beachfront property” with the improved engineering of the TDD technologies that use this unpaired spectrum. And, only 5G-cellular can access millimeter-wave bands opened for exclusive licensed use.
New technologies have also enabled greater spectrum reuse with multiple spatial channels enabling spectrum reuse within a cell site sector.
However, there is something of a definitional blur between what is a technological improvement and what is densification in the triumvirate. For decades, cell sectorization has been a kind of densification, but it is achieved without increasing the number of cell sites. With most cell sites already sectorized by 2012, it is a moot point whether sectorization contributes to the densification increase in the triumvirate since then.
More significantly in the network capacity growth story, the spatial multiplexing that’s being achieved with MIMO beamforming over the last few years to create distinct “layers” might be interpreted as either technological improvement or densification. A MIMO layer refers to an independent data stream transmitted in a distinct channel between transmitter and receiver. Each layer can carry different information, and multiple layers can be transmitted simultaneously, exploiting spatial spectrum reuse to increase data throughput. It is sometimes claimed that spectrum reuse with MIMO beamforming enables the Shannon limit to be exceeded. This is impossible in the physics—that constraint still applies within any channel.
Nevertheless, MIMO beamforming can significantly enhance the capacity of wireless communication systems. Capacity gain achieved varies depending on the specific implementation and environment. However, in general, massive MIMO systems can improve the capacity of wireless networks by up to 10 times or even more. In addition to implementing massive MIMO in unpaired spectrum with TDD at 2.5GHz and above, this capacity booster is also now being implemented in paired spectrum with FDD in frequencies between 1 GHz and 2 GHz. The latter is particularly attractive in nations where some higher frequency bands have not yet been released for cellular.
Is massive MIMO an improvement in spectrum efficiency or is it densification? It doesn’t really matter either way how one defines things because the overall effect on the capacity increase is the same. A multiple of 10 multiplied by the more physical densification multiple of 6 calculated previously yields a total densification multiple of 60. That is in line with the figure of 56 projected by SK Telecom. However, I suspect SK Telecom expected more of the former with additional physical end points than a conception of what has become possible virtually with the latter. Much of the seminal research work in this field was published no earlier than 2013. It is only a minority of cell sites — principally in urban areas where throughput demands are highest — that massive MIMO has been deployed so far.
However, the distinction between physical densification and what can be done virtually with massive MIMO matters a great deal in implementation because of the very different costs. Increasing capacity where and when required with the addition of massive MIMO is a highly scalable and economic solution that is much cheaper than cell splitting or HetNets with the addition of small cells using mid-band or millimeter wave spectrum.
More bandwidth
The bonus of going to higher frequencies is that there is exponentially more bandwidth there than at lower frequencies. This might not be immediately apparent to all because electromagnetic spectrum characteristics, allocations and usage are always graphically depicted on a logarithmic scale. For example, there is 10 times more bandwidth around (i.e. within a few percent of) 6 GHz than there is around 600 MHz.
However, it has only been in the last decade that mid-band spectrum, at 2.5 GHz and above, has become most competitive and valuable in cellular. For example, while Sprint and Clearwire together had had more than 120 MHz nationwide bandwidth of 2.5 GHz of spectrum for rolling out WiMAX services from the late 2000s, technological and supply ecosystem support for this unpaired spectrum was poor then because all the mainstream cellular standards used paired Spectrum with FDD technology.
The chip and device supply ecosystem stepped up in response to widespread carrier demand for LTE-TDD (TD-LTE), significantly driven by the large Chinese domestic market from the end of 2013.
Nevertheless, Craig Moffett, a well-known telecom analyst, expressed skepticism about the value of Sprint’s 2.5 GHz spectrum less than ten years ago, including with the challenges associated with deploying it effectively.
More recently, TDD has been able to capitalize on other subsequent technological developments including massive MIMO to boost capacity and HPUE that improves coverage.
Propagation is key
Two major factors determine whether spectrum provides sufficient propagation to provide widespread network capacity increases economically: are frequencies low enough and is maximum transmission power high enough?
The sweet spot for network-wide deployment is with the full-power licensing of mid-band frequencies. These can have good enough propagation to provide full network coverage as well as sufficient bandwidth and suitability for use of massive MIMO to provide lots of additional capacity.
In discussions at a round table session at 5G Americas’ annual event for industry analysts recently, consensus among the cellular industry folk briefing us is that more spectrum needs to be exclusively licensed for full-power use. For example, the 7.125-8.4 GHz band, that can provide a significant proportion of the estimated 1.5-2.5GHz of bandwidth required for 6G, can be implemented with four times as many advanced antenna system elements per unit area than in the 3.5 GHz band. That can improve performance in various ways including compensating for higher propagation losses.
CBRS uptake is lackluster because of the low power and onerous out of-band interference limits. AT&T is seeking relocation of CBRS frequencies so the frequencies CBRS currently uses can be reallocated for full-power licensed cellular usage contiguously with other adjacent bands, namely the 3.45 GHz band and the C Band.
The uptake of LTE-LAA has also been lackluster due to power limitations for unlicensed device use.
WiFi is uneconomic for covering large areas outdoors. WiFi proponents continue to claim WiFi carries most data traffic, but this is within extremely limited geographic coverage like at home, in the office and in coffee shops. WiFi does a great job of providing enormous capacity over relatively small areas per access point. The main reason WiFi carries most data traffic is because most people wirelessly stream video to various devices in their homes .
Short-range cellular usage, including millimeter-wave where people gather in their droves, including sports stadiums, music arenas and transportation hubs is also very important, but these areas account for only a miniscule proportion of any nation’s landmass.
Economic trade-offs
Despite the high cost of exclusively-licensed full-power spectrum, this is generally cheaper than densification. Once obtained, for example, in auction, spectrum is also much quicker to deploy. US MNOs altogether spent $100 billion including clearing and relocation costs for the 280 MHz of bandwidth exclusively licensed in the 2021 C-band spectrum auction. This spectrum enables capacity to be gradually increased when and wherever required across an MNO’s existing network grid. Capacity can be further increased by adding massive MIMO selectively to those sites where high traffic demands warrant it.
GSMA asserts that the greatest economic benefit from the upper 6 GHz in all countries is where it is “assigned for licensed, macro-cell mobile with standard power levels.” New spectrum in the 7.125-8.4 GHz band would further provide MNOs with spectrum for 6G in bandwidth blocks from 100 MHz to 400 MHz.
The capital cost of deploying 5G network equipment is worthwhile because it enables network capacity growth by accessing spectrum that cannot not be used for 4G. It is also cost effective in substituting for previous generations of network equipment that tend to use spectrum less efficiently, for example, also in terms of power consumption.
Physical densification with addition of small cell sites will remain a costly means of increasing network capacity. Planning restrictions will continue to prevent or delay many prospective deployments. In addition to equipment and installation costs, sites that can serve public areas must usually be leased from tower companies or property owners. MNOs make adequate financial returns while paying rent for collocating at macro sites with typically more than one tenant obtaining coverage and usage for many subscribers over a wide area. On the other hand, the economic value of a small cell site is much lower. Some site owners, for example, municipalities leasing space on street furniture, seek rents that are disproportionate to that lower value, making small cell densification much less economic or prohibitively uneconomic.